Microencapsulation is a highly important tool for food process engineers. Protected during storage or processing, released at the right time and place, the encapsulated vitamins, enzymes, plant extracts, unsaturated fatty acids, flavors and aromas offer a unique approach for maintaining optimum quality and nutritive status. Encapsulation also constitutes an attractive tool for enabling new properties to usual materials, or as a biocatalyst immobilization system to process food in a safer and more efficient manner. The food encapsulation market is estimated to be worth $14.1 billion by 2025.
The Challenge
Microencapsulation is a process of packaging or covering small solid, liquid, or gaseous particles in a secondary material to produce a microcapsule, with a typical diameter ranging from 1 to 1000 mm. The types of encapsulated core ingredients are flavor agents (sweeteners, seasonings, and spices), lipids, enzymes, amino acids and peptides, vitamins, minerals, antioxidants, or soluble fibers. The encapsulant materials are typically carbohydrates, proteins, lipids, and waxes. The encapsulation coatings form a barrier around the core material to protect it from degradation or separate it from its surroundings during processing and storage (oxygen-sensitive flavor and color or highly reactive materials). The core material is ‘released’ when required in a controlled, precise, and timely manner: time-dependent, temperature change, moisture, pH, enzymatic, or mechanical rupture. Materials simulations can be of great relevance for the food encapsulation market because they can assess in silico the microcapsule properties, such as its structure and stability, the impact of the encapsulant material, and in turn, the performance and mechanisms of encapsulation. This can be obtained by creating the digital twins of encapsulated core ingredient and membrane of choice using coarse-grained molecular dynamics simulations, providing the advantage of simulating large systems at long time scales by reducing the number of degrees as compared to all-atoms models.
The Work
Coarse-grained molecular dynamics simulations with Martini force field were used to model the encapsulation of limonene in two membrane materials: pluronic L64 and calcium alginate using the LAMMPS plugin in MAPS. Structural analysis tools within the MAPS platform were used to characterize the surface and volume distribution of the micelles formed during the encapsulation process.
The Results
Pluronics are amphiphilic linear triblock copolymers consisting of two hydrophilic polyethylene oxide (PEO) blocks connected to a central hydrophobic polypropylene oxide (PPO) block. Their main applications are in cosmetics and medical and pharmaceutical industries as surface coating materials and drug/gene nanocarriers, exploiting the pluronics ability to self-assembled in polar solvents. Pluronic L64 consists of thirty PPO blocs connected on both ends to thirteen PEO blocks, i.e. (PEO)13-(PPO)30-(PEO)13. The limonene molecule was represented using two SC1 beads (-CH2-CH2-), one SC3 (-CH=C-CH3) for the aromatic ring, and a C2 bead for the -CH2=C-CH3 substituent. Four water molecules were mapped into one P4 bead.
The encapsulation process of 1 wt % of limonene and 10 wt % Pluronic L64 in water was simulated in an NPT-MD run at 300 K for 500 ns. Figure 1 shows the formation of large isolated micelles with a surface of 8000-14000 Å2 and a volume in the range of 140000-220000 Å3. The limonene core itself has a surface of 2500-4500 Å2 and a volume of 35000-55000 Å3. This results in an average diameter of limonene of ~50 Å.
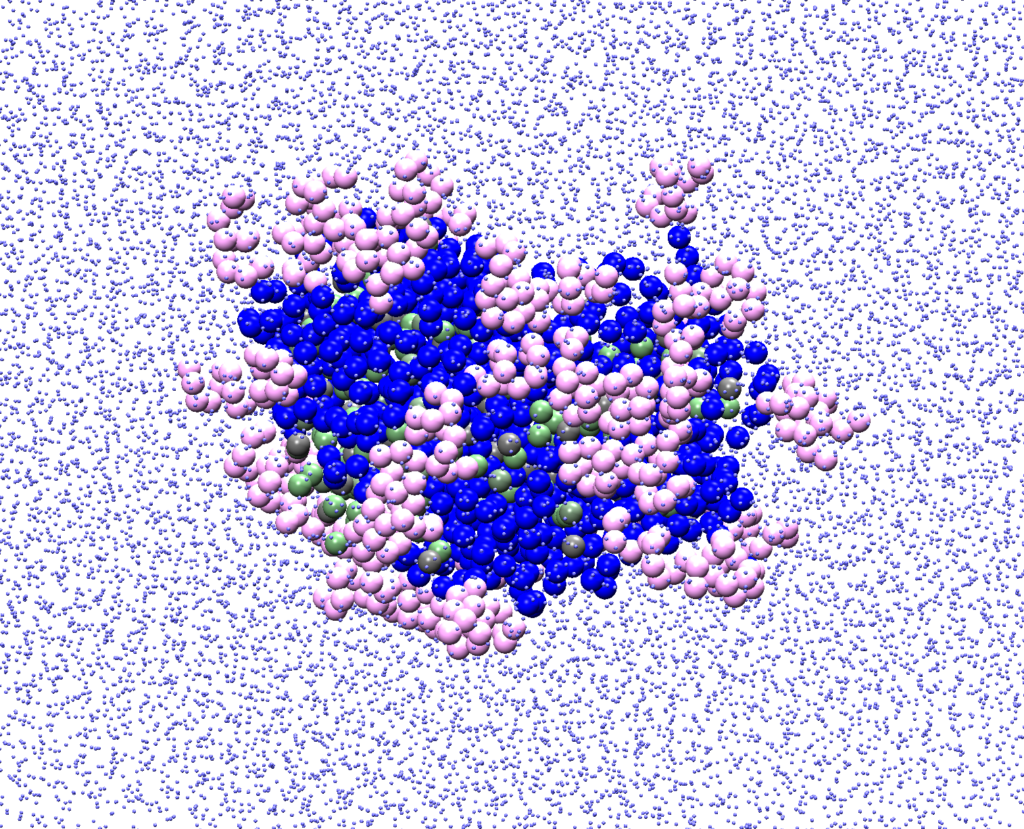
Figure 1: Encapsulation of limonene in a pluronic L64 membrane.
Similarly, an NPT simulation of 1 wt % limonene and 10 wt % calcium alginate in water was performed at 300 K for 500 ns. The results are depicted in Figure 2 showing the formation of rod-like connected micelles encapsulating smaller limonene cores (400-1900 Å2 surface and 4000-28000 Å3 volume), having a maximum diameter of 35 Å. The alginate creates a network connecting the limonene micelles and allowing for greater stabilization of the encapsulated product.
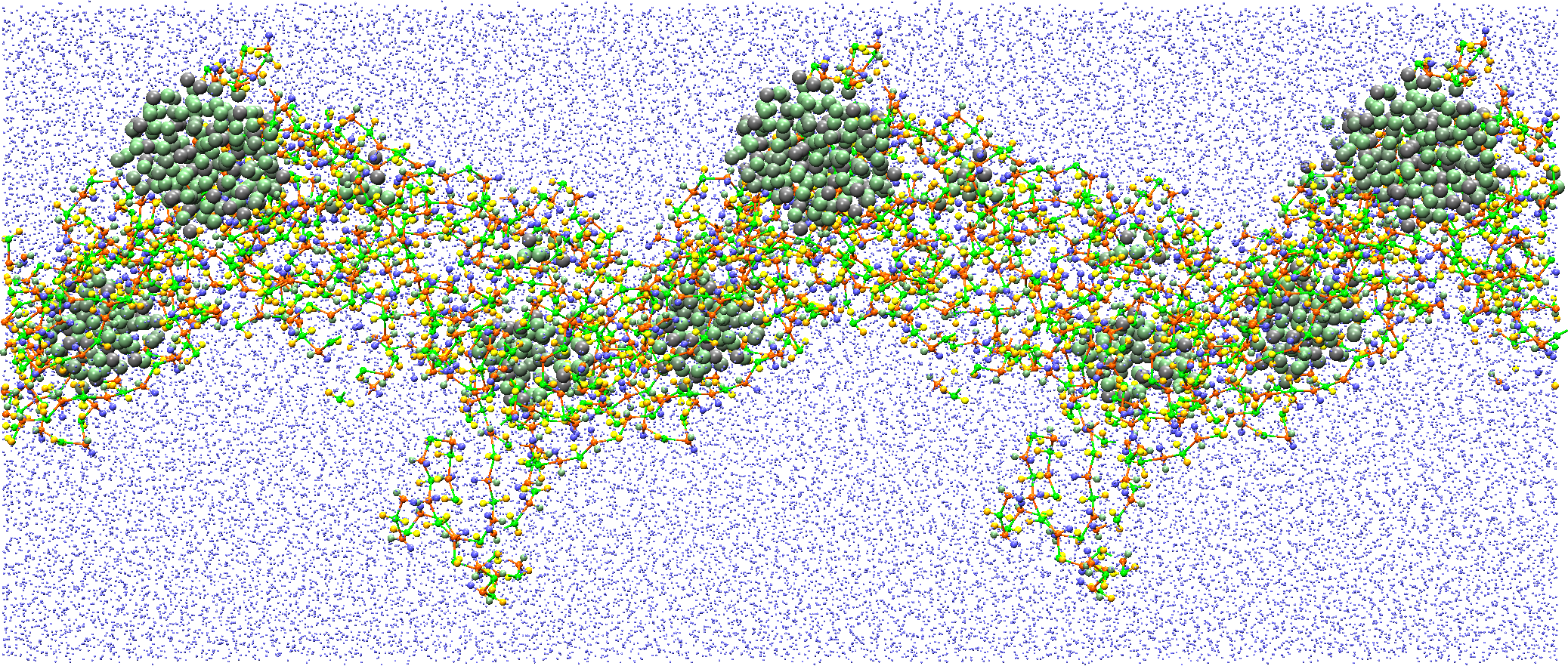
Figure2: Encapsulation of limonene in a calcium alginate membrane.
Coarse-grained molecular dynamics simulations and the technology deployed by SCIENOMICS allow assessing the structural stability and adaptability of the membranes to encapsulate food ingredients, providing guiding principles and design insights for the follow-up experimental synthesis.